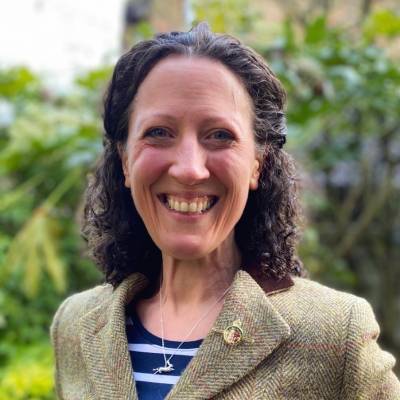
Sir John Hammond was a true pioneer, without whom we may never have been able to harness the myriad technologies that we now employ within livestock breeding. If we look back to the characteristic shapes and sizes of cattle, sheep and other animals in the early 20th century, it’s clear that considerable genetic changes have occurred over time with the advent of selective breeding. Given the current industry, consumer and policymaker focus on sustainable food production, it’s clear that we will have to adopt both existing and new technologies to continue to move forwards, yet it’s not always obvious how much of a contribution reproductive technology may play in sustainable systems.
Given the brilliance of Sir John Hammond, and the renown that he has within our industry, there is a certain irony in the fact that if you search for ‘John Hammond’ on popular internet search engines, the top results relate instead to another reproductive expert. Perhaps coincidentally (although the author suspects not), Michael Crichton, author of many best-selling books, chose the name John Hammond for the geneticist who managed to bring dinosaurs back to life in the book (and film) Jurassic Park. The resulting dinosaur appropriation of the entire theme park, with death and destruction trailing in their wake, was, of course, an entirely unsustainable consequence.
By contrast, the contribution made to the livestock industry by Sir John Hammond CBE FRS PhD, has greatly improved cattle industry sustainability over the past century. Sir John’s recognition of the timing of oestrus in cattle; classic studies in embryo survival and authorship of the first authoritative text on artificial insemination (AI) mean that he can truly be considered the father of modern animal physiology. Perhaps most importantly (at least with reference to this conference paper), he founded the British Cattle Breeders Club in 1946 and was an active member of the European Association of Animal Physiology during the early days of its inception in the early 1950s. It is interesting to note that when Sir John conducted his pioneering AI studies the work was considered socially unacceptable and as such, often had to be transferred overseas (Wilmot, 2007). Yet AI has a history that extends further back than we might suppose. For example, the first (albeit anecdotal) incidence of human AI is postulated to have occurred in 1461, through the actions of King Henry IV King of Castile, nicknamed ‘the impotent’ (Ombelet and Van Robays, 2015). The first documented human AI was undertaken in 1770, followed by successful AI of a springer spaniel in 1784. 150 years later, in the late 1930s, the applicability of AI to UK farming systems was doubted by many, citing concerns relating to livestock standardisation and a future lack of genetic diversity. Nevertheless, in 1943 Sir John headed the first Cattle AI centre based in Cambridge and, a mere eight years later, 20% of UK dairy cattle were served by AI (Wilmot, 2007). By 1958, AI was used in 58% of dairy cows and, almost a century later, AI has become the standard technology in dairy cattle breeding.
These dramatic gains in technology adoption have had considerable benefits to the rate of genetic gain within dairy and other livestock industries, yet we should not assume that we are now in a societal position where all technologies are automatically welcomed by the consumer. Scientific advances in human medicine, telecommunications, transport and other sectors have generally had a positive reception from consumers. However, a small yet insidious perception that technology use within food production, particularly technologies that have the potential to cause pain or distress to livestock, still exists for many consumers, with nostalgic views of farming garnering favourable opinions. This may be exemplified by the painting ‘The Farm’ by Alexis Rockman (2000). The artwork depicts changes in farm animal appearance and use from ‘traditional’ farming through to imagined future animals, including a six-winged, four-legged naked chicken and mouse with a human ear grown on its back. The date by which the future farm animals might have been created is not stated, yet it’s interesting to note that the artwork includes an image of an obese pig growing organs for human transplantation. Given recent media reports of a porcine kidney being successfully surgically attached to a human and apparently functioning normally (Roberts, 2021), this future vision may not be as far ahead as originally conceptualised. Concerns over technology use in all food production sectors will therefore remain a significant social sustainability issue going forwards.
Sustainability has become a surprisingly complex issue within food production, as although it appears to be a simple concept, the exact definition varies considerably according to the desires and perceptions of the beholder. To some, sustainable systems may conjure visions of organic, smallscale farms using native breeds; to others it means improving efficiency through large-scale, intensive production and making the best use of by- and co-product feeds. From a scientific perspective, the consensus definition is a balance between economic viability, environmental responsibility and social acceptability (United Nations, 2005), a balance that must be striven for in all livestock systems. One of the greatest sustainability issues that the cattle industry needs to overcome is the gap in knowledge and understanding between the producer and the consumer, which, when hitherto underknown practices are exposed, may lead to accusations that the farming industry lacks transparency. There is a significant difference between intentional misrepresentation versus failing to communicate every advance in farm management practices, yet communication must be improved for producers to gain the social license to operate in future (Capper, 2020).
Advances in information technology that allow us to find the answer to almost any question in a matter of moments, or to talk with colleagues thousands of miles away, also lead to a greater need to consider consumer views when adopting new or existing farm technologies, with the knowledge that these views may not accede with our own. For example, Pieper et al. (2016) surveyed the attitudes of German consumers to reproductive technologies used in farm animals and reported that the proportion of consumers viewing these practices in a negative light appeared to increase with the artifice or invasiveness of the practice. Consequently, 53% of consumers were opposed to the use of sexed semen, 58% to embryo transfer, 65% to fertility programmes and 81% to cloning. As with any consumer survey and as noted by the researchers, results may be biased by the question formatting. For example, the question ‘are you concerned about hormones being injected into dairy cows?’ would be expected to yield different answers to ‘what are your top 3 concerns about dairy farming?‘ Nevertheless, the results reported by Pieper et al. (2016), did reveal a general lack of awareness by consumers regarding the reproductive practices used on dairy farms, and therefore the need for improved outreach and education.
Meat labelling, until now, has largely been confined to marketing claims, nutritional information and badges from accreditation schemes, yet it seems likely that, in future, considerably more information will be available. It’s conceivable that consumers will be able to access sustainability information on a range of topics and metrics (e.g. carbon footprint, biodiversity, antimicrobial use, production system, etc) through traffic-light style colour codes, numerical indices or QR codes within in the next 5–10 years (Capper, 2020). This will have significant implications for the potential social acceptability of technologies used on farm, yet will also necessitate a greater technology adoption rate with regards to being able to measure, benchmark, compare and prove the effects of farm practices on sustainability metrics.
The focus of many sustainability efforts still remaining strongly upon greenhouse gas (GHG) emissions however, particularly in the wake of COP26. Although GHG emissions vary considerably across the globe, developed livestock industries have tended to reduce their emissions per kg of food produced through improvements in genetics, nutrition, health and management over the past century (Capper et al., 2009; Capper, 2011; Cady et al., 2013; Xin et al., 2013; Legesse et al., 2016; Capper and Cady, 2020; Ottosen et al., 2021). Perhaps the most extreme example in terms of productivity gains may be the USA, in which a wholescale move towards large-scale farming has conferred reductions in the GHG emissions per kg of milk by 63% between 1944 and 2007 and a further 19% between 2007 and 2017; per kg of beef by 18% between 1977 and 2007; per kg of pork by 35% between 1959 and 2009; and per tonne of eggs by 63% between 1960 and 2010 (Capper et al., 2009; Capper, 2011; Cady et al., 2013; Xin et al., 2013). The magnitudes of these gains could lead to the supposition that future gains may be capped given the biological limits that exist for growth rates, carcass sizes or milk yields and the eventual plateauing of efficiencies of scale. However, given that the USA-based dairy cow that holds the world record for 365-d milk production yielded 35,437 kg, it is clear that further genetic gains may still be available within dairy herds. Indeed, Shook (2006) reported that approximately 55% of the gains made in US dairy productivity between 1980 and 2006 were due to genetics, and it is logical to suppose that, given the highly integrated nature of monogastric production and supply chains, an even higher proportion of global pig and poultry efficiencies may have been conferred by improved genetic merit as discussed by Ottosen et al. (2020).
Beef production may be considered the exception to the rule, both in the USA and in the UK. Despite differences in scale, the industry structure is largely similar between the two countries, including a relatively limited uptake of reproductive technology compared to the dairy sector, which presents a considerable opportunity going forwards. Increased slaughter weight was one of the major driving factors behind the reductions in GHG emissions per kg HCW beef identified by Capper (2011) between 1977 and 2007, however, trends in UK beef cattle carcass weight reveal that gains have been moderate at best, with an increase of 27kg between 2003 and 2020 (an average of 1.6 kg/year; Statistica, 2021). This has partly been directed by laudable efforts to reduce suckler cow bodyweight and therefore maintenance nutrient costs and resource use. Given the additional difficulty of processing and marketing larger carcasses to UK consumers, increasing carcass weight is not a sustainable strategy for UK beef production.
The other force behind the US beef industry’s environmental improvements over time was increased daily liveweight gain (DLWG) that facilitated cattle being slaughtered at an average of 450 d in 2007 compared to 609 d in 1977. Age at slaughter has already been identified by Kamilaris et al. (2020) as one of the major determinants of production system efficiency in UK production and therefore an opportunity to cut both resource use and economic costs going forwards. At the simplest level, a reduction in slaughter age from, say, 24 mo to 21 mo, saves three months of pasture, water intake and GHG emissions. Given the gains in animal science knowledge over the past half century, it’s therefore somewhat surprising that the average slaughter age of UK cattle hovers between 24–26 mo of age. This is partially cultural – the traditional nature of British beef production mean that many producers sell cattle at a fixed point in the year rather than according to liveweight and condition. This was echoed by Barber (2018), who reported that few suckler producers regularly weighed their cattle, relying on sale or deadweight values to estimate performance. Nevertheless, there is a significant opportunity for improvement though cooperative purchasing of weighing equipment between farms, or investment in new digital technologies that will provide image-based estimates of weight and condition score and allow cattle to be sold at the optimum time.
The suggestion that DLWG should be increased to finish cattle earlier may be met by resistance and the argument that it is not possible without considerable supplemental feed and therefore increased environmental impacts. Yet, by contrast, a considerable number of producers are already finishing cattle off grass at 24 mo of age. This cannot necessarily be achieved on every operation across the UK and the associated economic viability is dependent on input costs, support payments and system resilience (Kamilaris et al., 2020; Rutherford et al., 2021), however, premiums are being paid by some processors to enable this level of performance. If appropriate breeding goals are identified to ensure that cows and calves can make the best use of the resources available; pasture and feed are managed efficiently and with due regard for optimal production; and livestock health is made a priority to avoid sub-clinical and clinical disease losses; then the national average age at slaughter may be reduced, leading to sustainability gains.
It’s rather a cliche to suggest that there are as many beef systems in the UK as there are beef farms, yet this aphorism contains a grain of truth. Debate therefore continues to rage as to the most sustainable breed for UK beef production systems. Although this discussion is likely to continue for decades, it is clear that the most sustainable breed is one that fits the opportunities and limitations of a specific farm and production system (Greenwood, 2021). It may therefore be time to recognise that although it’s vital to improve communication and cooperation throughout the UK beef industry, it has essentially become two sectors – based on either suckler cows or dairy beef – and the sustainability goals and outcomes of each system must be assessed accordingly.
Many processors and retailers have come to recognise the reductions in GHG emissions associated with producing beef from dairy calves, given that a considerable proportion of the dam’s environmental impacts can be allocated to milk production (Murphy et al., 2017). Dairy beef systems have considerable potential to improve environmental sustainability, especially if animals are reared and finished in either intensive or 24- mo systems, in addition to solving one of the major social acceptability issues of dairy production – the fate of dairy bull calves (Rutherford et al., 2021). Given the preponderance of AI and growing use of sexed semen in the dairy industry, there are also considerable opportunities to have a significant influence on the genetics of the resulting calves, while maintaining important dairy traits (Berry et al., 2019). The potential gains of this approach may be extended further by the use of assisted reproduction techniques and integrated dairy-beef programs similar to those described by Crowe et al. (2021) that make use of oocyte recovery and embryo transfer to ensure that dairy calf crops comprise only high genetic merit dairy heifers plus premium-quality beef calves.
Given the widespread media attention to GHG emissions from beef production, the suckler sector is likely to come under greater scrutiny going forwards and, as such, will need to be proactive in terms of demonstrating their sustainability benefits. As discussed by Wilkinson (2011); Hennessy et al. (2021) and Wilkinson and Lee (2018), both feed efficiency and land use for suckler beef production appears environmentally undesirable compared to cereal beef systems. However, the proportion of the land used for suckler production that could be used to produce human food and fibre crops is extremely low compared to other livestock sectors. Hence suckler beef has an important sustainability role in making efficient and sustainable use of the 60% of UK land that is unsuitable for anything but pasture (DEFRA, 2020), which should be promoted to the processor, consumer and policymaker. Nevertheless, this must be done in tandem with efforts to improve productivity through targeted breeding goals that allow producers to meet appropriate KPI (e.g. calves weaned at 50% of maternal bodyweight, high proportion of live calves born per 100 cows bred, pasture utilisation and stocking rates, age at slaughter, antimicrobial use, etc) while meeting processor specifications and maintaining excellent meat quality.
Ultimately, the sustainability of the UK beef industry may be improved by breeding and genetics, but only if three premises hold true. Firstly, appropriate breeding goals must be selected for the relevant systems, with due regard for current and future challenges within both beef and dairy production (Brito et al., 2021; Greenwood, 2021). These include (but are not limited to):
- A focus on feed efficiency to improve productivity, cut methane emissions and make best use of by-product feeds and forages that humans cannot or will not eat
- Meeting and exceeding sector specific KPI
- Engendering resistance to both endemic diseases and to other health challenges that may occur in future as a consequence of climate variability
- Enhancing meat quality and flavour parameters
- Harnessing the potential to enhance meat’s nutritional profile and market as a future functional food
- Accounting for other environmental metrics, e.g. water use, intra- and inter-species biodiversity, air pollution, etc.
Secondly, technology use should be encouraged and improved throughout the beef industry. This encompasses all types of technology, from the relatively prosaic (e.g. weighing cattle), to reproductive and other technologies that have existed for some time but have not been widely adopted (e.g. AI, sexed semen, embryo transfer); to new and innovative practices (e.g. genome editing, molecular breeding values) that are still under development (McFarlane et al., 2019; Terry et al., 2020). Crucially, these must be undertaken in combination with improved data collection, recording and benchmarking. This will be facilitated in future by electronic livestock ID and the development of platforms that allow different apps or services to be linked, thereby reducing both repetitive data inputs and inputting error, but must be initiated now to avoid a lag phase. Concurrent changes in farmer behaviour and on-farm practices may be achieved by greater use of peer-to-peer learning and discussion groups, as described by Morgans et al. (2019) when successfully evoking changes in antimicrobial use on dairy farms. Without clear data showing the gains made over time, it’s impossible to demonstrate the beef industry’s dedication to improving sustainability and therefore to mount a defence against the myriad challenges that are already building momentum. However, it is also essential that both the benefits of changes in management practice are clearly outlined to the producer. These may involve tangible environmental and economic gains such as described by Quinton et al. (2018) in their assessment of using AI to improve maternal traits in beef cows; may allow access to specific processors and retailers; or simply be associated with improved social acceptability (e.g. dairy bull calves being reared for beef rather than euthanised on farm).
Finally, improved communication both up and down the beef production, processing and supply chain is imperative. Processors and retailers are faced with the challenge of assessing the GHG emissions and other environmental metrics relating to their supply chain, and many will put policies, procedures and tools in place to source these data. These initiatives must be farm-focused, achievable and adoptable in order to be successful, and should be developed in consultation with farmers and allied industry. The ultimate final product is beef that is produced with a low environmental impact and conveys an excellent eating experience to a satisfied consumer. However, this relies upon upstream communication, with improved linkages needing to be formed between the processor or retailer and dairy and producers earlier in the chain. As discussed by Pritchard et al. (2021), data on ultimate end-product quality must therefore be fed back to dairy and suckler producers to allow them to make informed breeding decisions that enhance whole system sustainability.
References
Barber, B. (2018) Benchmarking beef suckler herds in practice: the initial steps. Livestock https://doi.org/10.12968/live.2018.23.3.124
Berry, DP., Amer, PR., Evans, RD., Byrne, T., Cromie, AR. and Hely, F. (2019) A breeding index to rank beef bulls for use on dairy females to maximize profit. J Dairy Sci, 102(11):10056–10072. https://doi.org/10.3168/jds.2019-16912
Brito, LF., Bedere, N., Douhard, F., Oliveira, HR., Arnal, M., Penagaricano, F., Schinckel, AP., Baes, CF. and Miglior, F. (2021) Review: Genetic selection of high-yielding dairy cattle toward sustainable farming systems in a rapidly changing world. Animal, 15:100292. https://doi.org/10.1016/j.animal.2021.100292
Cady, RA., Boyd, G., Wittig, L., Bryan, G., Holden, PJ, Sutton, AL. and Anderson, D. (2013) A 50-year comparison of the environmental impact and resource use of the U.S. swine herd: 1959 vs. 2009. Proceedings of the ADSA-ASAS Joint Annual Meeting, Indianapolis, IN, USA.
Capper, JL. (2011) The environmental impact of beef production in the United States: 1977 compared with 2007. J Anim Sci, 89:4249–4261. http://doi.org/10.2527/jas.2010-3784
Capper, JL. (2020) Opportunities and challenges in animal protein industry sustainability: The battle between science and consumer perception. Anim Front, 10(4):7–13. https://doi.org/10.1093/af/vfaa034
Capper, JL. and Cady, RA. (2020) The effects of improved performance in the US dairy cattle industry on environmental impacts between 2007 and 2017. J Anim Sci, 98(1):1–14. https://doi.org/10.1093/jas/skz291
Capper, JL, Cady, RA. and Bauman, DE. (2009) The environmental impact of dairy production: 1944 compared with 2007. J Anim Sci, 87:2160–2167. http://doi.org/10.2527/jas.2009-1781
Crowe, AD., Lonergan, P. and Butler, ST. (2021) Invited review: Use of assisted reproduction techniques to accelerate genetic gain and increase value of beef production in dairy herds. J Dairy Sci, 104(12):12189–12206. https://doi.org/10.3168/jds.2021-20281
DEFRA (2020) Farming Statistics – final crop areas, yields, livestock populations and agricultural workforce at 1 June 2020 United Kingdom. DEFRA, London, UK.
Greenwood, PL. (2021) Review: An overview of beef production from pasture and feedlot globally, as demand for beef and the need for sustainable practices increase. Animal, 15:100295. https://doi.org/10.1016/ j.animal.2021.100295
Hennessy, DP., Shalloo, L., van Zanten, HHE., Schop, M. and De Boer, IJM. (2021) The net contribution of livestock to the supply of human edible protein: the case of Ireland. The Journal of Agricultural Science 159(5–6):463–471. https://doi.org/10.1017/S0021859621000642
Kamilaris, C., Dewhurst, RJ., Vosough Ahmadi, B., Crosson, P. and Alexander, P. (2020) A bioeconomic model for cost analysis of alternative management strategies in beef finishing systems. Ag Sys, 180:102713. https://doi.org/ 10.1016/j.agsy.2019.102713
Legesse, G., Beauchemin, KA., Ominski, KH., McGeough, EJ., Kroebel, R., MacDonald, D., Little, SM. and McAllister. TA. (2016) Greenhouse gas emissions of Canadian beef production in 1981 as compared with 2011. Animal Production Science 56(3):153–168. https://doi.org/10.1071/AN15386
McFarlane, GR., Salvesen, HA., Sternberg, A. and Lillico, SG. (2019) On-farm livestock genome editing using cutting edge reproductive technologies. Front Sust Food Sys, 3 https://doi.org/10.3389/fsufs.2019.00106
Morgans, L., Reyher, KK., Barrett, DC., Turner, A., Bellini, J., Elkins, P. and Clarke, T. (2019) Changing farmer and veterinarian behaviour around antimicrobial use. Livestock 24(2):75–80. https://doi.org/10.12968/ live.2019.24.2.75
Murphy, B., Crosson, P., Kelly, AK. and Prendiville, R. (2017) An economic and greenhouse gas emissions evaluation of pasture-based dairy calf-to-beef production systems. Ag Sys, 154:124–132. https://doi.org/10.1016/j.agsy.2017.03.007
Ombelet, W. and Van Robays J. (2015) Artificial insemination history: hurdles and milestones. Facts Views Vis Obgyn 7(2):137–143.
Ottosen, M., Mackenzie, SG., Filipe, JAN., Misiura, MM. and Kyriazakis, I. (2021) Changes in the environmental impacts of pig production systems in Great Britain over the last 18 years. Ag Sys, 189:103063. https://doi.org/10.1016/ j.agsy.2021.103063
Ottosen, M., Mackenzie, SG., Wallace, M. and Kyriazakis, I. (2020) A method to estimate the environmental impacts from genetic change in pig production systems. The International Journal of Life Cycle Assessment 25(3):523–537. https://doi.org/10.1007/s11367- 019-01686-8
Pieper, L., Doherr, MG. and Heuwieser, W. (2016) Consumers’ attitudes about milk quality and fertilization methods in dairy cows in Germany. J Dairy Sci, 99(4):3162–3170. https://doi.org/10.3168/jds.2015-10169
Pritchard, TC., Wall, E. and Coffey, MP. (2021) Genetic parameters for carcase measurements and age at slaughter in commercial cattle. Animal, 15(2):100090. https://doi.org/10.1016/ j.animal.2020.100090
Quinton, C., Amer, P., Kirk, T. and Wall, E. (2018) Predicted economic and greenhouse gas benefits from using improved maternal genetics in UK beef cattle Proceedings of the World Congress on Genetics Applied to Livestock Production No. 11. p 364.
Roberts, M. (2021) US surgeons test pig kidney transplant in a human. https://www.bbc.co.uk/news/health-58993696 Accessed January 21st 2022.
Rockman, A. (2000) The Farm. Exit Art Gallery, New York City, NY, USA
Rutherford, NH., Lively, FO. and Arnott, G. (2021). A review of beef production systems for the sustainable use of surplus male dairy-origin calves within the UK. Front Vet Sci, 8:635497– 635497. https://doi.org/10.3389/ fvets.2021.635497
Shook, GE. (2006) Major advances in determining appropriate selection goals. J Dairy Sci, 89:1349–1361. https://doi.org/10.3168/ jds.S0022-0302(06)72202-0
Statistica (2021) Average carcass weight of slaughtered prime cattle in the United Kingdom (UK) from 2003 to 2021. https://www. statista.com/statistics/298332/carcase-weight-of-slaughtered-prime-cattle-in-the-united-kingdom.uk/ Accessed 3rd January 2022.
Terry, SA., Basarab, JA., Guan, LL. and McAllister, TA. (2020) Strategies to improve the efficiency of beef cattle production. Canadian Journal of Animal Science 101(1):1–19. https://doi.org/10.1139/cjas-2020-0022 United Nations (2005) 2005 World Summit Outcome.
United Nations Publications, New York, NY, USA. Wilkinson, JM. (2011) Re-defining efficiency of feed use by livestock. Animal, 5:1014–1022. https://doi.org/10.1017/S175173111100005X
Wilkinson, JM. and Lee, MRF. (2018) Review: Use of human-edible animal feeds by ruminant livestock. Animal, 12(8):1735–1743. https://doi.org/10.1017/S175173111700218X
Wilmot, S. (2007) From ‘public service’ to artificial insemination: animal breeding science and reproductive research in early twentieth-century Britain. Studies in History and Philosophy of Science Part C: Studies in History and Philosophy of Biological and Biomedical Sciences 38(2):411–441. https://doi.org/10.1016/ j.shpsc.2007.03.007
Xin, H., Ibarburu, M., Vold, L. and Pelletier, N. (2013) A Comparative Assessment of the Environmental Footprint of the U.S. Egg Industry in 1960 and 2010. Egg Industry Center, Des Moines, IA