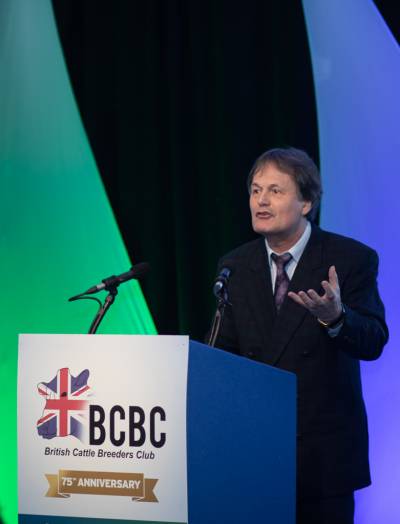
The livestock industry in particular beef and dairy production is under a substantial pressure to achieve net zero greenhouse gas (GHG) emissions. The main GHG responsible for this is methane emissions from rumen fermentation of feed. Methane emissions from agriculture is a highly potent greenhouse gas (GHG) estimated to be 27.2 times higher than that of CO2 (IPCC, 2021). Based on a recently released UN report, methane emissions are responsible for about 30% of the global anthropogenic emissions since pre-industrial times. Of these methane emissions, 32% are estimated to be from global agriculture mainly from cattle (United Nations Environment Programme and Climate and Clean Air Coalition, 2021). In the UK, 60% of the agricultural GHG emissions expressed as CO2 equivalent are reported to be due to methane emissions (Brown et al., 2021). These emissions are estimated to be more than 80% from ruminal fermentation of feed especially from cattle. Therefore, reduction in methane emissions from cattle is one main aim to achieve net zero emissions from cattle production.
I think it is a combination of strategies necessary to achieve this net zero aim such as dietary intervention (e.g. seaweed, 3-NOP), change in husbandry and management systems (e.g. optimisation of days to slaughter) and breeding. Breeding for reduced methane emissions per kg beef (methane intensity) can be achieved by selection on performance traits such as feed efficiency, growth rate, longevity, animal health, fertility, mature cow size, gestation length and age at first calving. This strategy is known as indirect selection on traits linked to methane mitigation per kg product, mainly on those presently selected for in most breeding programmes. An increase in selection pressure on these indirect traits genetically correlated to methane emissions in the breeding goal or the creation of a specific index for climate smart efficient cattle using these traits could be used to focus selection more on reduction in methane emissions per kg product. A study in dairy by de Haas et al. (2021) predicted that selection over 32 years based on present traits in the Dutch breeding goal is expected to result in 24% reduction in GHG emissions per kg product. Although a recognisable contribution, the time to achieve the reduction in GHG emissions per kg product is very long so that direct selection for methane mitigation is required to achieve the UK net zero emission’s goal by 2050.
Direct selection
Direct selection based on measured or predicted methane emissions is only effective if there are firstly large differences between beef cattle in methane emissions per kg dry matter intake under consideration that the animals have eaten the same diet, are housed, and raised under the same management conditions and are from the same breed. Secondly, these differences in methane emissions between beef cattle need to be linked to the animal genome and thus inherited from the parents to their progeny. Thirdly, the cost associated with measuring or alternatively predicting methane emissions of individual cattle have to be at a magnitude so that this breeding strategy is cost-effective.
Difference of animals in methane emissions
At SRUC, we have measured methane emissions of individual beef cattle in respiration chambers and observed large differences in methane emissions between them. Figure 1 illustrates that the low methane emitting cattle showing approximately half of the methane emissions per kg dry matter intake than those of high emitters. This is an excellent basis for selection for reduction in methane emissions if these differences are inherited, i.e., transmitted by genes from the sire to its progeny.
Figure 1: High differences in low and high methane emitting cattle (same trial, breed, diet, feed Intake, rearing & husbandry; Roehe et al., 2016)
Animal genetic impact on methane emissions
In an earlier study, we found that there are significant differences between sire progeny groups in methane emissions (Roehe et al., 2016) indicating that there are AI bulls inheriting low methane emissions. Recently, we estimated based on the SRUC population, which consist of Aberdeen Angus and Limousin rotational crosses, Charolais crosses and Luing, a moderate heritability of 0.33 for methane emissions (Martínez-Álvaro et al., 2022). The magnitude of this heritability is similar to that, e.g., of growth rate and feed efficiency, indicating that selection for methane emissions will be as successful as those traits, most likely even higher due to the large difference in methane emissions between animals within breed. Our estimated heritability for methane emissions is in the range of those reviewed by Brito et al. (2018), where 60 heritablity estimates for methane emissions of different studies in beef and dairy cattle as well as sheep were analysed. Recently, Hossein-Zadeh (2022) reported a review of heritability estimates of different methane emissions traits in different dairy cattle populations which are further confirming that the cattle genome influences the production of methane of methanogenic archaea in the rumen.
Measuring of methane emissions
SRUC has an excellent GreenCow facility, where we measure methane emissions individually in respiration chambers. These chambers are the gold-standard for measuring methane emissions from ruminants. They have to be large for cattle to not alter their behaviour and thus methane emissions (Picture 1). Methane emissions measured in respiration chambers are mostly used in experiments to identify the genetic impact of this trait, to test the effect of diets to mitigate these emissions or for validation of proxy measurements to predict methane emissions. We used the respiration chambers mainly to identify the accuracy of rumen microbiome information to estimate methane emissions from beef cattle. This was necessary because it continues large scale genetic evaluation of cattle, the use of respiration chambers to measure methane emissions is too costly and therefore we investigated to use the rumen microbiome as cost-effective proxy trait for estimating methane emissions of each individual cattle.
Rumen microbiome composition to determine methane emissions
The rumen contains a very dense microbial ecosystem comprising of different bacteria, protozoa, fungi and methanogenic archaea. This microbial ecosystem within the rumen is essential in cattle due to its ability to convert by cattle enzymes indigestible fibrous plant material (e.g. grass) into absorbable nutrients used to produce high quality beef. As a result of unnecessary excess hydrogen produced during microbial conversion of feed, the rumen microbial archaea population produces the by-product methane, which is expelled through mouth and nose into the atmosphere. Several studies identified microbiome biomarkers as accurate proxies for estimation of methane emissions, most likely due to the fact that the synthesis of methane from ruminants is a direct consequence of the microbial metabolism in the rumen (Roehe et al., 2016; Auffret et al., 2018; Martínez-Álvaro et al., 2020). Rumen microbiome also plays a key role in cattle growth performance (Myer et al., 2017; Lima et al., 2019), since approximately 70% of their energy requirements are from volatile fatty acids produced by microbial fermentation in the rumen (Bergman, 1990). In addition, microbial protein produced in the rumen is the major protein source of cattle (Strom and Øskov, 1984); and many microbiome-derived metabolites act as regulatory signals in the microbiome-gut-brain axis (Carabotti et al., 2015) which may influence feed intake and feeding behaviour (Sommer and Bäckhed, 2013). Therefore, microbial biomarkers have also been used to predict feed efficiency, feed intake and growth (Lima et al., 2019). The influence of host genetics on the rumen microbiome, firstly suggested by Weimer et al. (2010) after a ruminal microbiome exchange, is now widely recognised not only in cattle (Roehe et al., 2016; Li et al., 2019; Zhang et al., 2020) but also in many other species (Bergamaschi et al., 2020; Chen et al., 2018; Tabrett and Horton, 2020). This opens up the opportunity to use the composition of the rumen microbiome for prediction of complex traits such as methane emissions and feed efficiency, not only for their phenotypic prediction but also for estimation of their breeding values.
Selection of the most informative microbiome information
For the same animals as shown in Figure 1, we observed that the abundances of the microbial genes (here for example the microbial gene fwdA that catalyse the first step of methane metabolism) are even more sensitive to identify low and high emitting cattle as shown in Figure 2. This was the first indication that the abundances of microbial genes are highly informative for prediction of methane emissions.
Figure 2: Low methane emitters showed substantially lower relative abundances of the microbial gene fwdA involved in methane metabolism in comparison to the high methane emitters (same trial, breed, diet, feed Intake, rearing & husbandry; Roehe et al., 2016)
However, there are further other factors to be considered that the abundances of the microbial genes can be used as efficient breeding criterion. The microbial gene must be present in the vast majority of animals in the population (i.e., be part of the core microbiome), exhibit wide phenotypic variation among animals, be heritable, and be genetically correlated with traits of interest, here methane emissions or feed efficiency. Our research as collaboration of SRUC, Genus plc and the University of Edinburgh revealed that approximately 30% of the composition of the functional core microbiome is influenced by host genetics, with heritabilities ranging from 0.15 to 0.66 (Martínez-Álvaro et al., 2022). Similar ranges of heritabilities were reported for the abundance of microbial community (i.e. the abundances of the identified specific microbes) in dairy cows in the range from 0.08 to 0.62 (Saborío‐Montero et al., 2020; Cardinale and Kadarmideen, 2022). The magnitude of genetic correlation of the microbiome composition depends strongly on the extent to which the trait of interest is part of the heritable microbiome and involved in metabolites required for production of methane. Martínez-Álvaro et al. (2022) found that among the heritable part of the rumen microbiome, a greater number of microbial genes had a stronger genetic association (genetic correlations with absolute values in the range from 0.59 to 0.93) with methane emissions than the abundance of specific microbes (115 vs. 29). This indicates that the composition of the microbial genes in the rumen is the most informative microbiome characteristic to breed low methane emitting cattle without its extremely costly measurement of methane emissions. These microbial genes carried by bacteria, protozoa and fungi were involved in specific metabolic processes associated with the essential microbial fermentation of feed to provide most of the energy and protein for the animal to produce meat. Based on our results, we proposed a selection index based on the abundances of the 30 most informative microbial genes to reduce methane emissions, in the following referred to as microbiome-driven breeding strategy.
Potential selection response
Our research as collaboration of SRUC with Genus plc and the University of Edinburgh has shown that the use of the abundances of the 30 most informative microbial genes in the microbiome-driven breeding strategy was highly efficient. When rumen microbiome-driven breeding is used intensively for methane mitigation in a cattle population, we predict based on data from experiments carried out at the SRUC Beef Research Centre, a reduction in methane emissions of up to 17% per generation. The microbiome-driven breeding strategy uses genomic selection to estimate the breeding values of methane emissions and could therefore result in a reduction of the generation internal to 2.5 years and thus result in an annual reduction in methane emissions by up to 7% per year. Microbiome-driven breeding can also be used to simultaneously improve the efficiency of cattle converting feed to meat and thus reducing the carbon footprint of beef and increasing the profitability of production (Lima et al., 2019). Preliminary analysis of the SRUC data suggest that selection on the abundances of 30 specific microbial genes genetically linked the feed conversion ratio can improve feed conversion ratio by up to 15% per generation. In addition, abundances of newly identified microbial species as obtained by Stewart et al. (2019) based on metagenomic sequences of ruminal microbial content from SRUC experimental cattle, could be used in microbiome-driven breeding to further reduce methane emissions or to improve animal health characteristics (e.g. prevention of ruminal acidosis) associated with the rumen microbiome. Our research also suggests that microbiome-driven breeding and dietary intervention are additive mitigation strategies and therefore can be efficiently combined to reduce methane emissions from cattle.
Conclusions
Microbiome-driven breeding is a highly promising new technique to produce climate smart efficient beef cattle. However, as any selection strategy, it needs a specific breeding programme where AI is highly present, cattle are genotyped using a SNP chip and rumen samples are taken from individual animals to determine the rumen microbiome. As our research has shown, if these requirements are provided, large changes in methane mitigation and improvement of feed efficiency can be achieved to obtain climate smart efficient cattle.
References
Auffret, M.D., Stewart, R., Dewhurst, R.J., Duthie, C.-A., Rooke, J.A., Wallace, R.J., Freeman, T.C., Snelling, T.J., Watson, M., Roehe, R., 2018. Identification, Comparison, and Validation of Robust Rumen Microbial Biomarkers for Methane Emissions Using Diverse Bos Taurus Breeds and Basal Diets. Front. Microbiol. 8. https://doi.org/10.3389/fmicb.2017.02642
Bergamaschi, M., Maltecca, C., Schillebeeckx, C., McNulty, N.P., Schwab, C., Shull, C., Fix, J., Tiezzi, F., 2020. Heritability and genome-wide association of swine gut microbiome features with growth and fatness parameters. Sci. Rep. 10, 1–12. https://doi.org/10.1038/s41598-020-66791-3
Bergman, E.N., 1990. Energy contributions of volatile fatty acids from the gastrointestinal tract in various species. Physiol. Rev. https://doi.org/10.1152/physrev.1990.70.2.567
Brito, L.F., Schenkel, F.S., Oliveira, H.R., Cánovas, A., Miglior, & F., 2018. Meta-analysis of heritability estimates for methane emission indicator traits in cattle and sheep. World Congr. Genet. Appl. to Livest. Prod. 700.
Brown P, Cardenas L, Choudrie S, Del Vento S, Karagianni E, MacCarthy J, Mullen P, Passant N, Richmond B, Smith H, Thistlethwaite G, Thomson A, Turtle L, W.D., 2021. UK Greenhouse Gas Inventory 1990 to 2019: Annual Report for submission under the Framework Convention on Climate Change. Department for Business, Energy & Industrial Strategy.
Carabotti, M., Scirocco, A., Maselli, M.A., Severi, C., 2015. The gut-brain axis: Interactions between enteric microbiota, central and enteric nervous systems. Ann. Gastroenterol. 28, 203–209.
Cardinale, S., Kadarmideen, H.N., 2022. Host Genome–Metagenome Analyses Using Combinatorial Network Methods Reveal Key Metagenomic and Host Genetic Features for Methane Emission and Feed Efficiency in Cattle. Front. Genet. 13, 1–12. https://doi.org/10.3389/fgene.2022.795717
Chen, C., Huang, X., Fang, S., Yang, H., He, M., Zhao, Y., Huang, L., 2018. Contribution of Host Genetics to the Variation of Microbial Composition of Cecum Lumen and Feces in Pigs. Front. Microbiol. 9, 1–13. https://doi.org/10.3389/fmicb.2018.02626
de Haas, Y., Veerkamp, R.F., de Jong, G., Aldridge, M.N., 2021. Selective breeding as a mitigation tool for methane emissions from dairy cattle. Animal 15, 100294. https://doi.org/10.1016/j.animal.2021.100294
Hossein-Zadeh, N.G., 2022. Estimates of the genetic contribution to methane emission in dairy cows: a meta-analysis. Sci. Rep. 12, 1–10. https://doi.org/10.1038/s41598-022-16778-z
IPCC, 2021. IPCC Sixth Assessment Report (AR6) Global Warming Potentials -.
Li, F., Li, C., Chen, Y., Liu, J., Zhang, C., Irving, B., Fitzsimmons, C., Plastow, G., Guan, L.L., 2019. Host genetics influence the rumen microbiota and heritable rumen microbial features associate with feed efficiency in cattle. Microbiome 7, 1–17. https://doi.org/10.1186/s40168-019-0699-1
Lima, J., Auffret, M.D., Stewart, R.D., Dewhurst, R.J., Duthie, C.-A., Snelling, T.J., Walker, A.W., Freeman, T.C., Watson, M., Roehe, R., 2019. Identification of Rumen Microbial Genes Involved in Pathways Linked to Appetite, Growth, and Feed Conversion Efficiency in Cattle. Front. Genet. 10, 1–18. https://doi.org/10.3389/fgene.2019.00701
Martínez-Álvaro, M., Auffret, M.D., Duthie, C.-A., Dewhurst, R.J., Cleveland, M.A., Watson, M., Roehe, R., 2022. Bovine host genome acts on rumen microbiome function linked to methane emissions. Commun. Biol. 5, 350. https://doi.org/10.1038/s42003-022-03293-0
Martínez-Álvaro, M., Auffret, M.D., Stewart, R.D., Dewhurst, R.J., Duthie, C.-A., Rooke, J.A., Wallace, R.J., Shih, B., Freeman, T.C., Watson, M., Roehe, R., 2020. Identification of Complex Rumen Microbiome Interaction Within Diverse Functional Niches as Mechanisms Affecting the Variation of Methane Emissions in Bovine. Front. Microbiol. 11, 1–13. https://doi.org/10.3389/fmicb.2020.00659
Martinez-Alvaro, M., Mattock, J., Weng, Z., Dewhurst, R.J., Cleveland, M.A., Watson, M., Roehe, R., 2022. Part of the functional rumen core microbiome is influenced by the bovine host genome and associated with feed efficiency, in: Proc. of Teh 12th World Congress on Genetics Applied to Livestock Production. Rotterdam, The Netherlands.
Myer, P.R., Freetly, H.C., Wells, J.E., Smith, T.P.L., Kuehn, L.A., 2017. Analysis of the gut bacterial communities in beef cattle and their association with feed intake, growth, and efficiency. J. Anim. Sci. 95, 3215–3224. https://doi.org/10.2527/jas2016.1059
Roehe, R., Dewhurst, R.J., Duthie, C.-A., Rooke, J.A., McKain, N., Ross, D.W., Hyslop, J.J., Waterhouse, A., Freeman, T.C., Watson, M., Wallace, R.J., 2016. Bovine Host Genetic Variation Influences Rumen Microbial Methane Production with Best Selection Criterion for Low Methane Emitting and Efficiently Feed Converting Hosts Based on Metagenomic Gene Abundance. PLoS Genet. 12. https://doi.org/10.1371/journal.pgen.1005846
Saborío‐Montero, A., Gutiérrez‐Rivas, M., García‐Rodríguez, A., Atxaerandio, R., Goiri, I., López de Maturana, E., Jiménez‐Montero, J.A., Alenda, R., González‐Recio, O., 2020. Structural equation models to disentangle the biological relationship between microbiota and complex traits: Methane production in dairy cattle as a case of study. J. Anim. Breed. Genet. 137, 36–48. https://doi.org/10.1111/jbg.12444
Sommer, F., Bäckhed, F., 2013. The gut microbiota-masters of host development and physiology. Nat. Rev. Microbiol. 11, 227–238. https://doi.org/10.1038/nrmicro2974
Stewart, R.D., Auffret, M.D., Warr, A., Walker, A.W., Roehe, R., Watson, M., 2019. Compendium of 4,941 rumen metagenome-assembled genomes for rumen microbiome biology and enzyme discovery. Nat. Biotechnol. 37, 953–961. https://doi.org/10.1038/s41587-019-0202-3
Strom, E., Øskov, E.R., 1984. The nutritive value of rumen micro-organisms in ruminants. Br. J. Nutr. 52, 613–620. https://doi.org/10.1079/bjn19840128
Tabrett, A., Horton, M.W., 2020. The influence of host genetics on the microbiome. F1000 Res. 9, 1–9.
United Nations Environment Programme and Climate and Clean Air Coalition, 2021. Global methane assessment: Benefits and Costs of Mitigating Methane Emissions. Nairobi, United Nations Environment Programme.
Weimer, P.J., Stevenson, D.M., Mantovani, H.C., Man, S.L.C., 2010. Host specificity of the ruminal bacterial community in the dairy cow following near-total exchange of ruminal contents. J. Dairy Sci. 93, 5902–5912. https://doi.org/10.3168/jds.2010-3500
Zhang, Q., Difford, G., Sahana, G., Løvendahl, P., Lassen, J., Lund, M.S., Guldbrandtsen, B., Janss, L., 2020. Bayesian modeling reveals host genetics associated with rumen microbiota jointly influence methane emission in dairy cows. ISME J. 14, 2019–2033. https://doi.org/10.1038/s41396-020-0663-x
Conference Photography Credit @Jenny Wood Photography