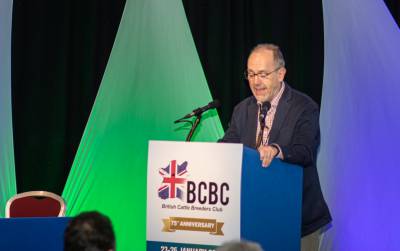
Food Systems Context
Our farming and food systems have responded remarkably well to feeding a rapidly growing population over the last century. As part of this response, the wider availability and affordability of animal-sourced foods (ASF) has contributed to food and nutritional security in many parts of the world. However, our farming and food systems face further challenges still. Continuing population growth is a major driver of these challenges - the population topped 8 billion recently and is set to approach 10.5 billion by the end of this century (UN, 2022). But it is not just about feeding more people, changing dietary preferences add to the challenge. Consumption of ASF has grown disproportionately over the last few decades (e.g. over a 5-fold increase in meat consumption in 60 years; Alexandratos and Bruinsma, 2012; Our World in Data, 2022) and consumption is expected to continue to rise, especially in lower and middle income countries. In some respects, the wider availability and affordability of ASF is a success story, but ASF can have both positive and negative health impacts. Even modest amounts of ASF can contribute to the wellbeing of children and other nutritionally vulnerable groups in some of world’s poorest settings (Grace et al., 2018). However, high consumption of some ASF has been linked to a number of diseases. (More broadly, our food systems and diets need to change globally if we are to tackle the ‘triple burden’ of malnutrition: obesity, micronutrient deficiency and hunger, and their health consequences.) ASF are also usually more resource intensive than many other foods, which exacerbates the effect that our food systems have on natural habitats and biodiversity.
Climate change poses twin challenges for our food systems. On the one hand we must reduce the contribution food systems, especially ASF, make to greenhouse gas (GHG) emissions - around a third of all emissions come from food systems (e.g. Crippa et al., 2021). On the other hand, our systems must adapt to unavoidable climate change – with temperature rises, changes in rainfall, and more frequent extreme events challenging current food production systems in many regions. For livestock, the biggest impacts are on feed availability and quality, the growing risk of heat stress in many parts of the world, and new disease risks (CCAFS, 2022). Climate change is becoming an increasingly important driver of food insecurity globally. The number of people living in acute food insecurity had been falling in the early years of this century, but has begun to rise again as a result of protracted conflict in several parts of the world, climate-related natural disasters, the covid pandemic (FAO, 2021), and the recent steep rise in food prices. (Our work in the Jameel Observatory https://jameelobservatory.org/ is seeking to improve anticipatory action to climate induced food shocks among pastoralists in East Africa, whose livelihoods depend on cattle and other livestock, and who are one of the groups most vulnerable to climate change.)
Livestock production – especially the dramatic growth in production globally – contributes to many of these food system challenges. As a result, the debate on the future of livestock production is often extremely polarised. However, as well as being part of the problem, livestock systems need to be part of the solution too. So, where does beef fit into feeding the world? In the rest of this paper, I will briefly review beef production’s ‘balance sheet’ or scorecard when it comes to sustainability, before outlining some of the targets for innovation – especially in breeding – that can help increase sustainability and thus support a long term positive contribution. The local and global challenges are interconnected, so I touch on both.
Beef’s sustainability ‘balance sheet’
In examining beef’s balance sheet, it helps to consider three pillars of sustainability: economic, social and environmental, though they overlap (see longer discussion in Simm et al., 2021). The beef sector’s major economic importance (around US$ 400 billion globally) is an important asset. However, the sector also has significant liabilities arising from its contribution to the challenges outlined above. As for the social dimension, beef is a valued dietary component for many, and an important source of dietary protein, energy and highly bioavailable micronutrients. Cattle also produce important co-products, including manure, and in many parts of the world transport and draught power, as well as being important cultural and capital assets. Livestock as a whole contribute to the livelihoods of around 1.7 billion poor people, the majority of whom are women (FAO, 2019). However, high consumption of red meat is believed to be associated with a higher incidence of some diseases (Dietary Guidelines Advisory Committee, 2020), and livestock farming and ASF are associated with some zoonotic diseases (diseases that can be transmitted to humans from other animals). There are public concerns over animal welfare in some beef production systems. With respect to the environmental pillar of sustainability, some beef systems, including well-managed grazing systems, locally and globally, support biodiversity and carbon sequestration. De Oliveira Silva et al., 2017, showed that restoring degraded pastures in Brazil could reconcile competing goals of livestock production with reduction of deforestation and GHG emissions. Such restoration is now a key part of Brazil’s international commitment to GHG reduction. Many beef systems make use of ‘low opportunity cost’ feed sources that cannot be consumed directly by humans (grassland, co-products from crop or other food production etc.). Beef breeds and the variation within them are important genetic resources, and part of agricultural biodiversity. However, the expansion of cattle production globally contributes to the loss of vital natural habitat, both directly and via land use for feed production. And lastly, ruminants in general and cattle in particular make a significant contribution to GHG emissions, especially via methane from the rumen.
Targets for improving sustainability
Optimal performance in sustainable systems
Despite the scale of the challenges, there are many opportunities to increase the sustainability of beef production. The overarching aim could be defined as to breed and manage for optimal performance in economically, socially and environmentally sustainable systems. While the specific challenges and priorities will vary from country to country, there will be common themes. Economic priorities usually include profitability, productivity and efficiency but, depending on the country and context, cattle may be valued mainly as financial and cultural assets. There is a particular need to improve productivity in regions or systems where this is low, yet environmental impact per unit of food produced is relatively high – so called sustainable intensification. Social priorities include the contribution of cattle farming to livelihoods; the affordability of the end products; animal welfare and the wider ‘social licence’ of systems, including the technologies used. Key environmental issues include GHG emissions; carbon sequestration potential; the use of land, water and other natural resources, and the circularity of systems; and impacts on nature and biodiversity, whether by sparing or sharing land for nature. Improvements in livestock breeding, nutrition, health and management can make major contributions to many of these priorities. For example, Capper (2011) compared the resource use of US beef production in 1977 and 2007. Beef produced in 2007 required 69.9% of the animals, 81.4% of the feed, 87.9% of the water and 67% of the land, while producing 81.9% of the manure, 82.3% of the methane, and 88% of the nitrous oxide, with a carbon footprint 16.3% lower than that of beef produced in 1977, as a result of such improvements. We need better metrics and tools to measure and manage system performance on this broad set of social, economic and environmental criteria (see e.g. https://www.trinityagtech.com/).
Low opportunity cost feed
‘Meat: the Four Futures project’ (https://tabledebates.org/meat) suggests four alternative future scenarios for meat, based on Garnett (2015): no meat, alternative meat, efficient meat and less meat. While improving resource use efficiency will usually be beneficial in futures involving meat production, it will be difficult for ruminants to compete with monogastric livestock on cost of production, resource use efficiency and GHG emissions, because of fundamental differences in their biology, including their reproductive rates. Ruminants fare better when considering a broader set of criteria, including their ability to use low opportunity cost feed sources and, in well-managed systems, their ability to support biodiversity. Van Zanten et al. (2018) showed that livestock’s ability to use co-products and other low opportunity cost feeds means that arable land use is minimized when between 16 and 40% of the human diet comes from animal sourced protein not, as many assume, with no ASF. In my view it is important that future breeding programmes and production systems support rather than dilute this ‘USP’ of ruminants e.g. by evaluating the genetic merit of animals in relevant systems. New livestock feed sources – such as insects, algae, novel microbial proteins - could also help reduce competition for land capable of growing human food directly.
Reducing methane emissions
A key priority for ruminant systems must be to reduce methane emissions. A range of studies has investigated the ability of dietary changes, feed additives, vaccines and breeding approaches to do so. The use of feed additives for housed cattle and breeding approaches are probably most likely to have impact in the short term (see Simm, 2021; Simm et al., 2021; Defra et al., 2022). Breeding for improved productivity already delivers indirect benefits in terms of GHG emissions per unit of product. Very rough calculations based on the resource use changes reported by Capper (2011) in the US, typical contributions of breeding to these system level improvements (Simm et al., 2021), comparative rates of genetic gain in US and UK beef breeds (Amer et al., 2015), and the contribution of productivity gains to GHG emission intensity (GHG per unit product) in other species (Simm, 2021) suggest that cumulative annual gains of 0.3-0.4% are probably being achieved now. So, reductions of the order of 8-9% in GHG emission intensity could be achieved by 2050, the intended date for achieving ‘Net Zero’ carbon in the UK and other countries. This could be enhanced further – probably doubled - by including selection on breeding values for emissions measured directly or via proxy measurements. There appears to be genetic variation among cattle in the population of rumen microbes they host, and so the levels of methane produced. This offers a particularly promising approach for future breeding schemes which could further increase gains (see Martínez-Álvaro et al., 2022, and Roehe, this conference). Provided that there are no unintended consequences of selection for reduced emissions, with faster rates of genetic improvement, rapid uptake of new technologies to assist breeding for reduced methane, and faster and wider penetration of improved breeding stock into commercial beef herds, reductions of up to a third in GHG emissions intensity through breeding could be feasible by 2050 in the UK. Similar results should be achievable in countries with effective improvement programmes targeting emissions. We need better tools to allow both breeders and their customers to identify, and be credited in the supply chain for producing, better breeding stock.
Effective breeding programmes
With one or two notable exceptions, rates of genetic improvement in key traits in UK beef breeds lag behind those in the US and Australia (Amer et al., 2015). There are historical, and both herd and industry structural reasons for this, but there has never been a more urgent need to overcome these to achieve sector-wide improvement in resource use efficiency and environmental impact. This needs more effective breeding programmes, better and more data on key performance attributes, and wider recognition of the ability of genetic improvement to make meaningful changes in performance over time. The work of the Irish Cattle Breeding Federation (https://www.icbf.com/) over the last 20 years or so is an exemplar of what can be achieved in terms of co-ordinated, industry-wide, comprehensive recording, use of new data, new genetic technologies and modern genetic evaluation methods. And there are examples too of good practice in the UK in some breeds and supply chains.
Genomic selection has started to revolutionise livestock breeding since it was first applied over a decade ago. It involves selection of breeding stock based on the use of genome-wide genetic markers, usually alongside conventional performance records (Simm et al., 2021), to identify individuals and families carrying markers identified in previous studies as being associated with traits of interest. Genomic selection can allow earlier identification of the best breeding stock, and also allow selection for traits that are difficult or expensive to measure in practice – like feed intake, methane emissions, maternal performance and disease resistance – once markers have been identified that are associated with these traits. Genomic selection is superseding progeny testing in dairy cattle breeding schemes in many countries, as it allows earlier and more accurate estimation of genetic merit of bulls. Beef breed structures and breeding practices mean that the benefits are likely to be lower in beef cattle, but worth pursuing (Amer et al., 2015), as some breeds are now doing.
Advances in statistical, genetic and reproductive technologies will continue to offer new opportunities. For instance, gene editing can allow targeted beneficial changes in traits of interests –especially those controlled by one or a small number of genes, including polling and resistance to some diseases.
Clarity of roles and breeding goals
It has long been recognised that the low output of meat per breeding female per annum compared to other more prolific farmed species limits the efficiency of beef systems, and so too the GHG emission intensity of beef. Using animals produced as a co-product of the dairy sector is one way to improve resource use efficiency and GHG intensity, as the inputs to the breeding herd can be shared between milk and meat outputs (e.g. Poore and Nemecek (2018) reported a 60% lower GHG intensity for dairy beef).
Within the specialised beef sector, choice of appropriate breeds and crosses, to optimize cow size and other key lifetime performance attributes, is crucial to system efficiency. It is also important to have clarity over the roles and breeding goals of different beef breeds or lines within them for use in dairy and beef herds. As several key terminal sire and maternal traits are antagonistic, failure to distinguish roles when making breeding decisions leads to inefficiency. In Britain and Ireland a focus on terminal sire traits in dual purpose and maternal breeds or lines has led to deterioration in maternal traits in many. For instance, Byrne (2018) estimated that annual gains in terminal traits in Ireland worth €33 masked a deterioration in maternal traits of €25 per annum.
Conclusion
Our food and farming systems have responded remarkably well to the challenge of feeding a growing global population over recent decades. We are now facing even more complex and interconnected challenges that need systemic responses. Livestock systems contribute to these challenges but are also an important part of the solution. Reducing greenhouse gas emissions from beef systems is a key target, but not the only one! Beef production has a mixed balance sheet or scorecard when it comes to sustainability, with a range of economic, social and environmental assets and liabilities. It is becoming increasingly important to enhance the assets – and seek more equitable distribution of the benefits globally - and to tackle the liabilities of beef systems. Effective breeding programmes produce cost-effective, cumulative and, over time, very significant changes in performance, and so need to be a key part of this response. There is a need and an opportunity to improve the effectiveness of beef breeding programmes both in countries with a long history of genetic improvement, like the UK, and especially in those countries where it has not been widely used, productivity is low, and environmental impact per unit of food produced is relatively high. Beef will continue to make an important contribution to food and nutritional security for many, especially so if beef breeders and producers, and other key actors in the supply chain, embrace the changes needed. While science and technology can make major contributions in this quest, it seems unlikely that they alone can meet the challenge of equitably feeding 10.5 billion people well without further damaging the natural systems on which we all depend. This will need social, economic and political innovation and interventions too.
References
Alexandratos, N and Bruinsma, J (2012) World agriculture towards 2030/2050: the 2012 revision (Vol. 12, No. 3). FAO, Rome: ESA Working paper. Available at: http://www.fao.org/docrep/016/ap106e/ap106e.pdf
Amer, P, Byrne, T, Fennessy, P, Jenkins, G, Martin-Collado, D and Berry, D (2015) Review of the genetic improvement of beef cattle and sheep in the UK with special reference to the potential for genomics. Report for EBLEX. Available at: 74404-Review-of-the-genetic-improvement-of-beef-cattle-and-sheep-in-the-UK-Final-report-140515.pdf
Byrne, T, Amer, P, Kirk, T, Hely, GF and Cromie, A (2018) 20 years of genetic improvement and Sheep Ireland genetics conference: ‘Sustainable Farming – Progress through Genetics’ 5 December 2018. Available at: https://www.icbf.com/wp/wp-content/ uploads/2018/12/Amer_20-years-of-ICBF.pdf
Capper, J L (2011) The environmental impact of beef production in the United States: 1977 compared with 2007. Journal of Animal Science 89: 4249-4261.
CCAFS (2022) Climate impact on production: Livestock. Available at: https://ccafs.cgiar.org/bigfacts/#theme=climate-impacts-production&subtheme=livestock
Crippa, M, Solazzo, E, Guizzardi, D et al. (2021) Food systems are responsible for a third of global anthropogenic GHG emissions. Nature Food 2, 198–209.
Defra et al. (2022): Call for evidence on methane suppressing feed products. Available at: https://consult.defra.gov.uk/agriclimate/methane-supressing-feed-products/supporting_documents/Methane%20Suppressing%20Feed%20Products%20Call%20for%20Evidence.pdf
De Oliveira Silva, R, Barioni, L G, Hall, J A J, Moretti, A C, Fonseca Veloso, R, Alexander, P, Crespolini, M, Moran, D (2017). Sustainable intensification of Brazilian livestock production through optimized pasture restoration. Agricultural Systems. 153, 201-211.
Dietary Guidelines Advisory Committee (2020) Scientific Report of the 2020 Dietary Guidelines Advisory Committee: Advisory Report to the Secretary of Agriculture and the Secretary of Health and Human Services. Washington, DC: US Department of Agriculture, Agricultural Research Service.
FAO (2019) Animal Production. Available at: http://www. fao.org/animal-production/en/
FAO (2021) The State of Food Security and Nutrition in the World. Available at: https://www.fao.org/state-of-food-security-nutrition/2021/en/
Garnett, T (2015). Gut feelings and possible tomorrows: fcrn_gut_feelings.pdf (tabledebates.org)
Grace, D, Dominguez-Salas, P, Alonso, S, Lannerstad, M, Muunda, E, Ngwili, N, Omar, A, Khan, M and Otobo, E (2018) The influence of livestock-derived foods on nutrition during the first 1,000 days of life. ILRI Research Report 44. International Livestock Research Institute, Nairobi, Kenya. Available at: https://cgspace.cgiar.org/handle/10568/92907
Martínez-Álvaro, M, Auffret, M D, Duthie, C-A, Dewhurst, R J, Cleveland, M A, Watson, M, Roehe, R (2022). Bovine host genome acts on rumen microbiome function linked to methane emissions. Communications Biology 5, 350 (2022). https://doi.org/10.1038/s42003-022-03293-0
Our World in Data (2022) Global meat consumption. Available at: https://ourworldindata.org/grapher/global-meat-projections-to-2050
Poore, J and Nemecek, T (2018) Reducing food’s environmental impacts through producers and consumers. Science 360, 987–992
Simm, G (2021) Livestock breeding and genetics and greenhouse gas emissions. Farming for 1.5⁰. Independent Inquiry on Farming and Climate Change in Scotland. Available at: https://www.farming1point5.org/_files/ugd/a7353c_ec118306f9274232a71286814ba9013e.pdf
Simm, G, Pollott, G, Mrode, R, Houston, R and Marshall, K (2021) Genetic improvement of farmed animals. CABI, Wallingford, UK.
United Nations (2022) World Population Prospects. Available at: World Population Prospects - Population Division - United Nations
Van Zanten, HHE, Herrero, M, Van Hal, O, Röös, E, Muller, A, Garnett, T, Gerber, PJ, Schader, C and De Boer, IJM (2018) Defining a land boundary for sustainable livestock consumption. Global Change Biology 24: 4185-4194.
Photography Credit @Jenny Wood Photography